AMANDA, Light of my Ice...
An underwater telescope called AMANDA, frozen deep in Antarctic ice, peers down at ghostly neutrinos that pass through Earth from above the Northern Hemisphere. (See Neutrino Nomads) Since neutrinos hardly interact with matter at all, they are ideal carriers of astronomical information across the vast reaches of the universe, quite impervious to scattering by interstellar matter or bending by magnetic fields. The same properties, however, that let neutrinos travel here unobstructed—no charge and hardly any mass—also make them extraordinarily difficult to detect.
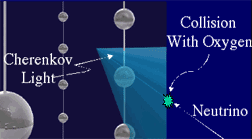
A neutrino interacts with the nucleus of an oxygen atom in the atmosphere, producing a muon. As the muon moves through Antarctic ice, it gives off Cherenkov radiation, shown in blue, which is detected by instruments in the spheres. As the muon moves through the array of these spheres, it creates a track that specifies its direction in space. (Image provided by the AMANDA-II Collaboration).
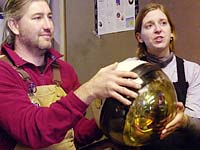
AMANDA researchers Darryn Schneider and Katherine Rawlins hold one of the light-detection modules (Photograph by Melanie Conner, courtesy of the National Science Foundation).
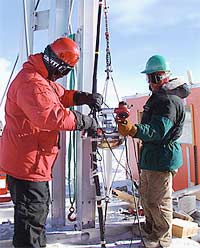
Two AMANDA investigators fasten a light detector to a cable, so the detector can be lowered into the hole melted in the Antarctic ice (Photograph by Josh Landis, courtesy of the National Science Foundation).
Neutrinos do interact occasionally with atomic nuclei, setting off a reaction that produces elementary particles called muons. The muons are emitted in the same direction in space as the neutrino was moving, so a measurement of the muon’s direction specifies the direction of the neutrino source. The muons move at the speed of light in vacuum, faster than the speed of light in the ice, and consequently they give off “Cherenkov” radiation in passing through the ice, in somewhat the same way that a moving ship makes a wake. So to find neutrinos, AMANDA detects light.
The light detectors, each about the size of a bowling ball, hang like beads on a string on long cables lowered into cylindrical holes in the ice. The holes are melted by a stream of hot water that produces a liquid cylinder about 50 cm in diameter and 2.5 km deep. Over the day it takes the water to refreeze, a cable, studded with as many as 42 detectors, is lowered into place.
The detectors form a three-dimensional array, located at the South Pole. As the muons pass through the ice, successive detections of the Cherenkov radiation yield a series of points along a line through the array and thus determine the muon’s path. Moreover, precise timing of the detector signals reveals which way along this path the muon moved, so the direction in space of the neutrino source can be specified.
A major complication in this experiment is the presence of a huge background of muons produced above Antarctica. When cosmic rays, mostly protons, interact with nuclei of nitrogen and oxygen in the atmosphere, muons are produced in large numbers and indeed are detected by AMANDA. To reject this background, precise timing, as mentioned above, reveals the direction of the muon track and rejects signals of muons moving downwards. Having the detectors point down helps, too, as does burying the detectors deep in the ice. As for muons produced above the northern hemisphere, the roughly 10,000 km of intervening rock filters them out as they pass through Earth to the South Pole.
Why build a neutrino telescope in such an inhospitable place as Antarctica?
- The solid ice provides a stable matrix for detectors.
- The ice is dark, clear, and free of radioactivity.
- Management of the experiment takes place on the solid surface—a much more stable platform than, say, a ship.
Research
An underwater telescope called AMANDA, frozen deep in Antarctic ice, peers down at ghostly neutrinos that pass through Earth from above the Northern Hemisphere. (See Neutrino Nomads ) Since neutrinos hardly interact with matter at all, they are ideal carriers of astronomical information across the vast reaches of the universe, quite impervious to scattering by interstellar matter or bending by magnetic fields. The same properties, however, that let neutrinos travel here unobstructed—no charge and hardly any mass—also make them extraordinarily difficult to detect.
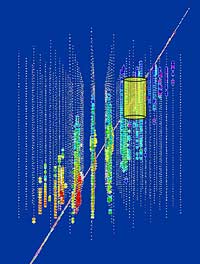
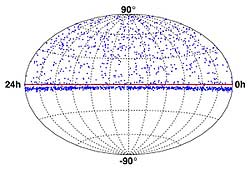
Direction of neutrino events detected by AMANDA-II, the second generation AMANDA instrument. 90 degrees indicates looking toward the North Pole, and the “equator” indicates the horizon. The red band below the horizon is the edge of the background from muons and atmospheric neutrinos produced above the South Pole. The data points in this map are attributed to neutrinos produced in the atmosphere above the northern hemisphere. (Image provided by the AMANDA-II collaboration)
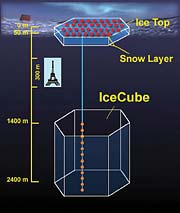
Layout of IceCube. (Image by Darwin Rianto, courtesy of Ice Cube Collaboration. This material is based upon work supported by the National Science Foundation under Grant Nos. OPP-9980474 [AMANDA] and OPP-0236449 [IceCube], University of Wisconsin-Madison.)
AMANDA functioned as a testbed and demonstrated the capability to detect neutrinos. In addition, to operate as a telescope it also had to accurately determine the direction of a neutrino’s path in space. To test its “pointing ability,” AMANDA was operated simultaneously with a much smaller and highly accurate particle detector. When the results were compared, the investigators found that AMANDA could correctly specify the muon path to within less than three degrees, sufficient accuracy for a neutrino telescope. The image of the neutrino sky shows AMANDA data.
With proof of concept established, the investigators are substantial enlarging AMANDA into an instrument, aptly named IceCube, with an array of detectors filling an entire cubic kilometer of ice. IceCube will consist of 4,800 detectors hung from 80 strings, in a volume large enough to detect neutrinos from distant astronomical sources, as shown in the two diagrams.
These astronomical neutrinos must be separated from the background of “atmospheric” neutrinos produced in secondary cosmic ray interactions, that is, from the decay of the products of cosmic ray-induced nuclear disintegrations. To distinguish between atmospheric and astronomical neutrinos, IceCube will rely on its greater size and efficiency to increase substantially the counts of detected neutrinos. The atmospheric neutrinos will be spread out uniformly, as in the above sky map, but the astronomical neutrinos will be concentrated around the direction of the neutrino source. IceCube must detect enough neutrinos so that an astronomical event in the distant universe will show up when the averaged background count is subtracted out.
In the past, as astronomers have extended their observations to microwaves, radio waves, and also to cosmic rays, each new kind of observation revealed fundamental processes at work in the universe or made possible the discovery of whole new classes of astronomical objects. Neutrino astronomy promises just such exciting results.
Links
University of Wisconsin
University of California, Irvine
Scientific American
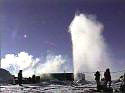
Drilling the cylindrical hole for a string of detectors by melting the ice with a stream of hot water. (Image provided by the AMANDA-II Collaboration)