Dark Matter
What is the universe made of? Solar absorption spectra showed that the Sun contains familiar elements, and spectra of stars in distant galaxies also revealed their presence. Even exotic objects such as as white dwarfs, supernovas, and neutron stars contain the familiar building blocks of matter. As for galaxies, up until about 30 years ago, they appeared to be composed of protons, neutrons and electrons--called baryonic matter--but now it appears most of the mass in galaxies, and clusters of galaxies, is made of something else, a hitherto unobserved kind of matter. This conclusion is supported by a confluence of findings ranging from galactic rotation to cosmology to gravitational lensing.
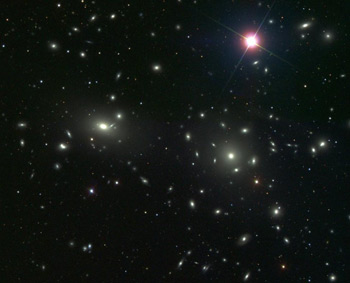
This is the Coma cluster. Studies of the motion of its outermost galaxies yield a much larger total mass than the mass that corresponds to the observed luminous matter. Image credit: Sloan Digital Sky Survey
Dynamics of Galactic Clusters
In 1938, Fritz Zwicky of Caltech found the first evidence of matter that interacts through gravitation but not through the electromagnetism, so it emits no light--hence the name "dark matter." Zwicky studied the statistics of the motion and location of galaxies in the Coma cluster, a gigantic system spanning more than 20,000,000 light-years (more than 200 times the size of a typical spiral galaxy). Zwicky assumed the cluster was spherically symmetrical, so the mutual gravitational force of all the galaxies attracts each one toward the cluster's center, and on the average the outermost galaxies ought to move according to Newtonian mechanics under the influence of a mass equal to the total mass of the cluster (see sidebar). By measuring the Doppler shifts of the spectral lines from these galaxies, Zwicky determined their average velocity. Independently, he measured the radius of the cluster. He calculated the total cluster mass necessary to produce the galactic motions that he had observed, and then, for comparison, he calculated the total mass based on the brightness of the galaxies. These two results were in violent disagreement--the mass based on the brightness of the galaxies was only about a 10th of the mass necessary to produce the observed motions.
The motion of galaxies in a spherical cluster is a central force problem, similar to the motion of the planets in circular orbits, but with randomness imposed by the mutual gravitational interactions of all the galaxies. The main idea can be seen easily from the mechanics of the circular motion of a single object in a circular orbit about a small central mass. Setting the centripetal acceleration equal to the gravitational acceleration gives
v2/r = GM/r2
where r = radius, v = velocity, M = cluster mass, and G = the gravitational constant. From this relationship, we can solve for M
M = v2r/G
or for v
v = (GM/r)1/2
Notice that the velocity decreases as the radius increases.
Dynamics of Galaxies
Nothing came of Zwicky's remarkable result for almost 40 years. Then, Vera Rubin and W.K. Ford stunned the astronomy community by reporting new spectroscopic measurements showing that stars in spiral galaxies rotate about galactic centers at about the same speed, independent of radial distance. Based on the brightness of the central "bulge," astronomers had assumed that most of the mass was concentrated there, where it would have produced a solar system-like distribution of velocities, decreasing with distance from the center. In the face of disbelief and criticism, Rubin and Ford stood their ground, and their results were in fact borne out by subsequent work in both spirals and ellipticals. Astronomers thus faced a stark choice: either Newton's law of gravitation did not hold over the size of the galaxy--about a hundred thousand light-years across--or galaxies contained substantial amounts of "dark matter," including in regions where very few stars were seen.
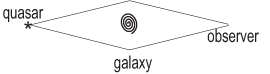
Schematic diagram of a gravitational lens
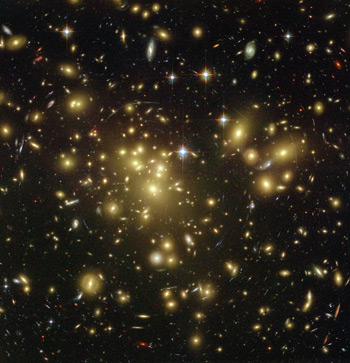
This image shows the galactic cluster Abell 1689, whose gravity distorts images of galaxies behind it (in the line of sight) through gravitational lensing. The mass obtained from lensing is far larger than the mass obtained from the luminous matter that is observed. Image credit: NASA, N. Benitez (JHU), T. Broadhurst (The Hebrew University), H. Ford (JHU), M. Clampin(STScI), G. Hartig (STScI), G. Illingworth (UCO/Lick Observatory), the ACS Science Team and ESA
Gravitational Lensing
An altogether different line of evidence for dark matter emerges from observations of gravitational lensing, the distortion in images of distant galaxies produced by the gravitational force of galaxies along the line of sight (see diagram). Look at the image of the Abell cluster of galaxies to see characteristic arcs produced by lensing. The mass distribution of the cluster was determined 1) by gravitational lensing and 2) by emitted light from the cluster, and the two masses were quite different, with the lensing mass corresponding to the results described above for galaxies and clusters of galaxies. A related technique called "weak lensing," which is a statistical study of tiny distortions of background galaxies seen in galactic surveys, provided further evidence.
Cosmology
Detailed studies of the microwave background radiation showed that the ordinary matter in the universe lacks the mutual gravitational force to produce the density fluctuations that led to the formation of stars and galaxies. To say this another way, the temperature and pressure of the ordinary matter were too high to enable the gravitational contraction of gas clouds to occur. Dark matter is therefore required for the existence of our universe.
So what are potential candidates for dark matter?
Protons, neutrons, and electrons (baryonic matter)
Atoms and molecules are excluded, because they would give off or absorb light. But cosmology severely constrains the possible amount of baryonic matter, because the relative abundance of hydrogen and helium is extremely sensitive to the amount of baryonic matter present in the early universe, which must be within a few percent of the matter that we now observe.
Non-barionic Matter
This category, which includes neutrinos, is the weakly interacting massive particles, or "WIMPs." These include
- hot dark matter (neutrinos, which move near the speed of light)
- warm dark matter (slower, but still relativistic)
- cold dark matter (nonrelativistic)
Some WIMPs predicted by the theory of supersymmetry are candidates for dark matter (This theory would double the number of particles by positing a counterpart, or "super partner," for each species of particle of ordinary matter).
This leaves physics in a quandary: Considering both dark matter and dark energy, which is quite a story in itself, the composition of the universe is as follows:
- 5% ordinary matter
- 25% dark matter
- 70% dark energy (and we have no idea what dark energy is)
Research
As mentioned above, the first evidence for dark matter came from a study of galactic clusters. In 2006, an investigation of the Bullet Cluster, which is composed of two colliding clusters of galaxies, provided important evidence for the existence of dark matter.
This cluster, about 3.4 billion light-years away, is unusual in that most of its matter consists of hot gases (see image).
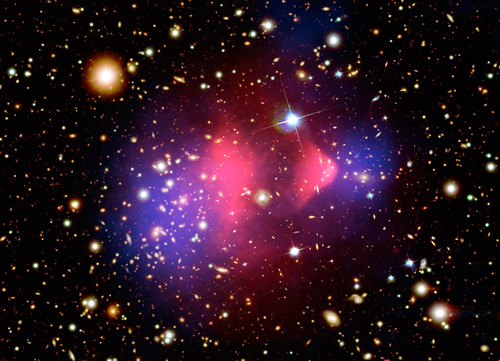
This image of the Bullet cluster shows a separation of dark matter from the luminous matter, with the dark matter shown in blue and the luminous matter shown in red. Image credit: X-ray: NASA/CXC/CfA/M.Markevitch et al.; Optical: NASA/STScI; Magellan/U.Arizona/D.Clowe et al.; Lensing Map: NASA/STScI; ESO WFI; Magellan/U.Arizona/D.Clowe et al.
Here's how it was investigated:
- Measurement of the emitted electromagnetic radiation indicated that most of the ordinary matter in the system was in the form of hot gases, which emit x-rays.
- Measurement of the distribution of x-ray emission produced the distribution of hot gases.
- A study of gravitational lensing (see previous section) determined the distribution of all the mass in the pair of colliding clusters.
During the collision, the colliding gases--ordinary matter-- interact through electrostatic forces between atoms and molecules, which slows their passage through each other. The dark matter in each galaxy, on the other hand, interacts only through the much weaker gravitational force, so its passage was not slowed by electrostatic interactions and over time it pulled ahead of the gas. The result of this investigation is shown in the image:
- blue regions show the distribution of the total mass, determined from gravitational lensing
- red regions show the hot gases, determined from x-ray emission
Since the red regions are separate from the blue regions, the vast preponderance of matter in the galaxy must be located in blue regions, since all matter in the cluster reacts gravitationally and thus contributes to gravitational lensing. This observation is taken to be the first direct evidence for dark matter, and this conclusion is now supported by a second example of dark matter-ordinary matter separation in colliding clusters (see Links).
Links
ESA: Clash of clusters provides new dark matter clue
NASA: "Five-Star" Rated Gravitational Lens
NASA: A Matter of Fact (Bullet Cluster)
NASA/ESA: Hubble Looks through Cosmic Zoom Lens
Physics World: Have We Seen the First Dark Galaxy?
UC Berkeley: Big Bang Nucleosynthesis
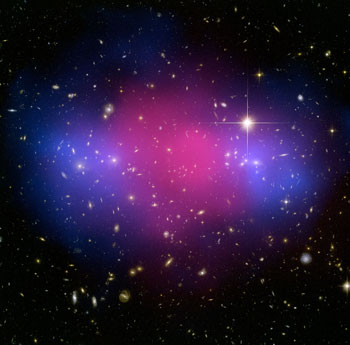
This pair of colliding clusters of galaxies provides confirmation for the separation of dark matter in ordinary matter observed in the Bullett cluster.
Image credit: NASA, ESA, CXC, M. Bradac (University of California, Santa Barbara, USA), and S. Allen (Stanford University, USA)