Pluto's Neighbor Could Float on Water
December 19, 2013
A recently discovered object in the Kuiper belt — Pluto’s cosmic neighborhood — has raised new questions about how planets form. Why? The incredibly light (for its size) object is the largest solid object ever found in our solar system that could float on water!1
Kuiper Belt Primer
Scientists once believed that Pluto was the only object in the Kuiper belt, but as technology improved, many more objects were found in the far reaches of our solar system. It may seem hard to believe, but scientists didn’t find another object in the Kuiper belt until 1992.
Since then, more than 1,300 objects have been observed.2 The increasing number of observed objects the size of Pluto and larger led astronomers to reclassify Pluto to a dwarf planet just a few years ago. More on that later.
The Kuiper belt occupies the space between 30 and 55 astronomical units away from the Sun. An astronomical unit (AU) is about equal to the mean distance from Earth to the Sun.
In terms of distances we are more familiar with, the Kuiper belt starts from Neptune’s orbit, about 4,487,936,130 km or 2,788,674,224 miles away (over a million times the distance between Los Angeles, CA and Washington DC). From there it goes out 3,739,946,775 km or 2,323,895,186 miles.
The distance across the Kuiper belt is about 871 thousand times the distance between Los Angeles, CA and Washington DC. The image below shows the known objects in the Kuiper belt in green (The orange colored objects are known as scattered disc objects or minor planets called centaurs).
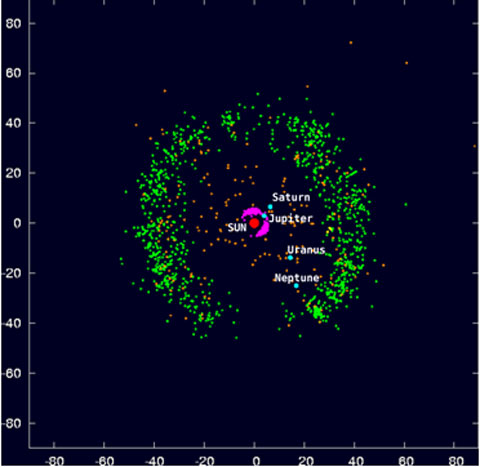
Image of the solar system, showing known Kuiper belt objects in green. The x- and y-axes measure astronomical units (1 AU equals about 93 million miles).
Image Credit: WilyD via Wikimedia Commons
Beyond the Kuiper belt lies the Oort cloud (5,000 to 10,000 AU), which contains icy objects that surround the outskirts of our solar system. In the Oort cloud, the Sun’s gravitational pull may not be as great as from other stars. It is believed that objects in the Oort cloud and the Kuiper belt have been there since the formation of our solar system about 4.6 billion years ago.2
Pluto's Demotion and Large Cosmic Neighbors
Pluto (with a diameter of 2300 km) was classified as a planet in our solar system until 2006. In 2005, after finding many Pluto-sized objects in the Kuiper belt, astronomers found Eris, an object originally measured to be slightly larger than Pluto, but recent measurements place it as slightly smaller than Pluto.
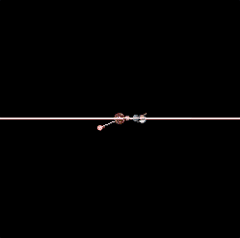
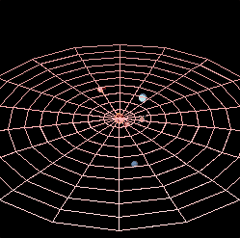
Pluto’s orbit showing side view along ecliptic and top view.
Image Credit: Lookang/Todd K. Timberlake
Eris has its own moon, orbits our Sun once every 560 years, and its orbit is between 38 and 98 AUs. Pluto, and its five known moons, orbits our Sun once every 248 years with an orbital distance between 29 and 49 AU.
The discovery of Eris required scientists to consider adding Eris as a tenth planet to our solar system, or reclassifying Pluto as a dwarf planet. In 2006 a group of astronomers decided that both Eris and Pluto (as well as an asteroid known as Ceres) would be classified as dwarf planets.
Current theory suggests that objects with a diameter of 350 km and smaller are very porous, and therefore have overall densities less than water. Scientists also believed that large objects, with diameters greater than 850 km, are denser than water.
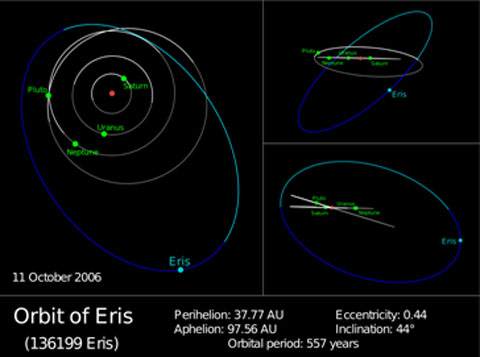
Eris’s orbit.
Image Credit: Orionist
For these large objects, smaller objects likely collect together (agglomerate). The gravitational pull of this larger object’s own mass on itself causes compression and the collapse of the empty space (pores) between the material — leading to densities greater than water.
Scientists have performed laboratory experiments on ice-rock models that support this theory. And all of this fits in with the idea of how small icy-rock planets form. Porous small objects attract conglomerate together. Their gravitational pull begins a compressive process.
As more objects combine, more compression occurs, increasing the density. However, objects would need to reach diameters of about 2700 km (the size of Triton, Neptune’s moon) before significant pressure-induced phase changes occur and even higher densities result to form dense icy-rock objects. If this is the process toward the creation of rock-rich dwarf planets like Pluto, then even more objects would need to combine.
So far there have been many measurements on Kuiper belt objects with diameters less than 350 km and greater than 850 km that support the theory above. If this theory is correct, the densities of mid-sized objects should be somewhere between those of small and big objects.
No object with a diameter between 350 km and 850 km (mid-sized) in the Kuiper belt had been measured until recently. The results will soon be published in the Astrophysical Journal, but can be seen in Reference 1.
The results show that the object known as 2002 UX25, has a diameter of 650 km and a density less than water. These measurements do not agree with the current theory of planetary formation.
Dr. Michael Brown, who calculated the inferred results, provides multiple reasons to explain why dwarf planets might still be created by the conglomeration and compression of multiple smaller objects, but he is not convinced of any of these reasons.1 In his paper, he writes: “The inferred low rock fraction of the 2002 UX25 system makes the formation of rock rich larger objects difficult to explain in any standard coagulation scenario.”1
He ends his conclusion with “We are left in an uncomfortable state of having no satisfying mechanism to explain the formation of the icy dwarf planets. While objects up to the size of 2002 UX25 can easily be formed through standard coagulation scenarios, the rock rich larger bodies may require a formation mechanism separate from the rest of the Kuiper belt.”1
Measuring Density Millions of Miles Away
So how do astronomers measure objects so far away and figure out the object’s mass and density?
First you need to know that gravity is a very weak force, and is proportional to the inverse distance squared. That means if there are two nearby objects, they are likely to affect each other more than two far away objects.

where G is a constant. One example of this is Earth and the Moon. The Earth’s proximity causes its gravitational pull to override the pull of other planets in the solar system.
Also remember that the force on the object equals its mass multiplied by its acceleration. For example, the gravitational force exerted by Earth on the Moon is equal to the Moon’s mass times the Moon’s acceleration (relative to Earth).
When the moon orbits around Earth with a relatively constant speed, then the acceleration points inward toward Earth. In this case, we can roughly equate the acceleration to the speed squared divided by the radius of the circle the object sweeps out. Putting this into our equation we have:

From this, we can see that we can measure Earth’s mass by knowing the measurements of the orbital speed of the Moon and the distance between the Moon and Earth.
Most objects don’t have a nice circular orbit, but an object’s location and how that is changing in time can be measured. From this information the speed and acceleration can be calculated.
The measurements for the mid-sized Kuiper belt object 2002 UX25 were made possible because it has a satellite (a moon). Observations of the system were made with the Hubble Space Telescope in 2005 with six follow up observations in 2006.1
Further observations were made at the Keck Observatory to clear up some ambiguities.1 Scientists then plugged the data into a mathematical model to map out 2002 UX25’s elliptical orbit. From this information the mass of 2002 UX25 is then determined.
The measurements of the sizes and densities of the objects are also described in reference 1. Thermal radiometry of the system was used to determine the diameter of the system using the Spitzer and Herschel Space Telescopes.1 Using visible and infrared wavelengths measurements with the Hubble Space Telescope and the Keck Observatory, the diameter of each object was approximated.
Density is just mass per volume. If we assume the object is spherical then the volume is proportional to the radius cubed of the object, and the density is given by:

What's Next?
The results of the measurements of object 2002 UX25 leads to many questions, and puts current theories of the formation of dwarf planets into question. Scientists will keep searching the Kuiper Belt for similarly sized objects for new clues.
References and Resources
1. Brown, M., The density of mid-sized Kuiper belt object 2002 UX25 and the formation of dwarf planets, preprint available at arXiv.org, 4 November 2013, Final print in the Astrophysical Journal Letters.http://arxiv.org/abs/1311.0553
2. Solar System Exploration, Kuiper Belt & Oort Cloud: Read More, NASA
http://solarsystem.nasa.gov/planets/profile.cfm?Object=KBOs&Display=OverviewLong
3. Cowen, R, Astronomers surprised by a large space rock less dense than water, Nature 13 November 2013.
http://www.nature.com/news/astronomers-surprised-by-large-space-rock-less-dense-than-water-1.14135
—Heide Doss