Through a Lens Darkly
About Negative Index of Refraction and the Superlens
What limits the sharpness of an image? The answer has to do with the wave nature of light. If you look at a bright light from a distance at night, and you bring your fingertips together so you see the light through the tiny space between them, you'll see bands. These bands are a diffraction pattern, caused by the interference of light at different parts of the opening between your fingertips. The closer you bring your fingers together, the more the bands spread out. (see photos).

This photograph shows the diffraction of red laser light as it passes through slits of different widths. Notice that the narrower the slit, the wider the diffraction pattern. (photo credit: Jaime Lush, Harvard University Science Center)
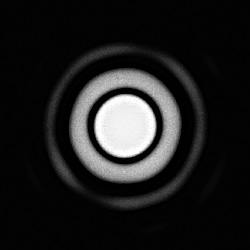
Diffraction pattern of a point source made by circular opening; a star imaged by a telescope makes a pattern like this. (photo credit: C.C. Jones, Union College, Schenectady, N.Y)
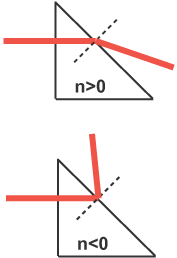
These drawings compare the path of light rays through a prism of glass (above) and of a negative-index-of-refraction material (below), both in air.
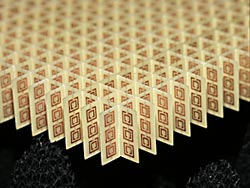
This drawing shows a metamaterial, composed of an array of wires and split metal rings. When illuminated by radiation with a wavelength much longer in the size of the wires and rings, this material has a negative index of refraction. (image courtesy of NASA)
Diffraction is caused when light strikes an edge and bends around it. When you take a photograph, the light passes through the circular opening of the lens, and the edge of the lens produces diffraction bands in the image. The photo shows diffraction rings—called Airy rings—produced by a circular aperture limits the fineness of detail, the resolution, that a lens can capture.
But not all the light that strikes a small object winds up in the diffraction pattern. Some of the light, called "near-field" or "evanescent," decays away so quickly with distance that it has all but disappeared within a wavelength or two, but this light contains information about the sub-wavelength detail of the object. In 2000, the English physicist John Pendry predicted that this "near-field" light could be imaged by a lens made of a material with a negative index of refraction.
The index of refraction specifies how a material bends light. For example, as light leaves a glass prism and enters air, the light beam bends as shown in the upper drawing, which corresponds to a positive index of refraction. But if the prism material has a negative index of refraction, the light would bend as shown in the lower drawing (see sidebar).
Negative index of refraction materials had been predicted back in the 1960s. In a theoretical study, the Soviet physicist Victor Veselago concluded that these materials would be consistent with the laws of physics, and would exhibit quite interesting behavior, including:
- reversal of the Doppler effect (light emitted by a receding object would be blue-shifted rather than red-shifted)
- backwards emission of Cherenkov radiation (radiation emitted by objects moving in a material at a speed faster than the speed of light in that material)
- backwards energy flow, compared with the direction that the light waves move.
Much later, in the 1990s, the English physicist John Pendry recognized that a lens need not be homogeneous, like glass or water, but rather could be a construction of different materials. The result, dubbed a “metamaterial,” refracted light, provided the constituents were all much smaller than the wavelength of the light. Typically, metamaterials are made from an array of wires and split metal rings, as shown in the photo. When light strikes this lens, the electrons move back and forth in the wires and rings, producing electric and magnetic fields.
In 2000, David Smith and a group at the University of California San Diego used millimeter-size constitutents to create a metamaterial lens that refracted a radar beam in a direction that corresponded to negative index of refraction. After some further theoretical work, the existence of these new materials was generally accepted.
Research
Veselago predicted that a rectangular solid with a negative index of refraction material would make an image of an object right in front of it, with the image created right behind. Pendry, many decades later, predicted that such a lens would be able to focus the near-field light into an image and avoid the diffraction limit. To catch the near field light, which decays away rapidly with distance from the object, the "superlens" would have to be pressed right up against the object.
Experimenters worked to make a negative index of fraction lens that would image the shortest wavelength light possible, to make the image as sharp as possible. Several groups succeeded in building a lens that worked at 1400 nm, about twice the wavelength of red light.
The discovery that a thin film of metal can have a negative index of refraction spurred physicists to fashion superlenses out of these films. In 2005, groups led by Richard Blaikie in New Zealand and also Xiang Zhang at the University of California, Berkeley succeeded in building a silver superlens that made an image with ultraviolet light (whose wavelength is shorter than that of visible light) and beat the diffraction limit. Those groups patterned gratings onto a mask, pressed the lens up against the mask, and created their images on photoresist, which undergoes a chemical change upon exposure to ultraviolet light. The image can be "seen" with an atomic force microscope.
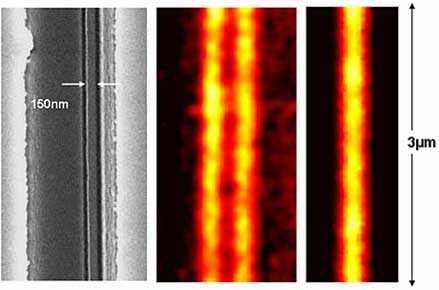
On the left is the object, two nanowires separated by 150 nanometers (nm). In the center is the image made by superlens, which clearly resolves the two wires, even though their separation was much less than the wavelength of the light used to make the image. On the right, without the superlens, the two wires cannot be resolved. (image credit: Zhang Lab, UC Berkeley)
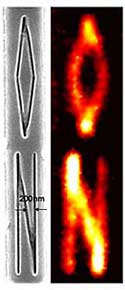
Here the superlens is imaging wires arranged into letters. The two arrows on the "N" indicate that the spacing there is 200 nm. (image credit: Zhang Lab, UC Berkeley)
Take a look at Zhang’s images. His objects were narrow lines--some were part of a grating, and others were fashioned into letters. The width of the letter strokes was 40 nm. Without the superlens, each stroke in the image had a width of about 320 nm, and with silver-film superlens in place, the width was under 90 nm--about a quarter of the wavelength of the 365 nm light used to make the image.
As far as actual applications are concerned, this revolutionary technology is limited, because the object, the lens, and the image are all right up against each other. But within the field of lithography, which is used to fabricate computer components, the superlens may make it possible to pack more components closer together on a silicon chip.
Negative index of refraction offers the prospect of other other exciting technologies as well. Pendry suggested that if an object were enclosed in a metamaterial, as shown in the diagram, light would be swept around the object, just as if it weren't there--a cloaking device. As physicists like to say, "Whatever is not forbidden is required."
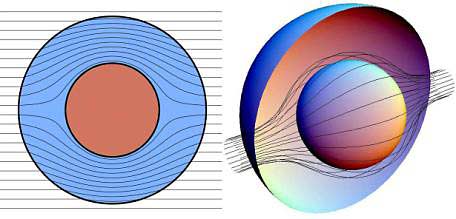
On the left is the object, two nanowires separated by 150 nanometers (nm). In the center is the image made by superlens, which clearly resolves the two wires, even though their separation was much less than the wavelength of the light used to make the image. On the right, without the superlens, the two wires cannot be resolved. (image credit: Zhang Lab, UC Berkeley)
Links
UCLA Galactic Center Group
National Radio Astronomy Observatory
Chandra X-ray Observatory
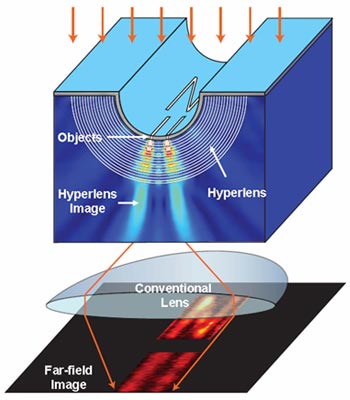
A schematic drawing of the superlens created by the Zhang group at UC Berkeley. (image credit: Zhang Lab, UC Berkeley)