Lithium-ion Batteries
Delivering a Charge
Lithium-ion batteries already power your cell phone and your laptop, and they may soon power your car. But what exactly are these batteries, and what makes them so much better than everyday alkaline batteries?
To answer this question, it's important to understand how batteries work. A battery is a device that stores electrical energy and can then deliver that energy through an easily controlled electro-chemical reaction.
A battery is usually composed of a series of cells that produce electricity. Each cell has three essential components: the anode, the cathode, and the electrolyte. When the anode and cathode are connected by an electrical conductor like a wire, electrons flow from the anode through the wire to the cathode, creating an electrical current, while the electrolyte conducts positive current in the form of positive ions, or cations. The materials used for each of these components determine the battery's characteristics, including its capacity—or total amount of energy it can deliver—and its voltage—or the amount of energy per electron. Imagine that a battery is like a tank of water being drained by a hose. The volume of the tank is the capacity of the battery, and the pressure in the hose is its voltage.
The anode and cathode materials are chosen so that the anode donates electrons, and the cathode accepts them. The tendency of a material to donate or accept electrons is commonly expressed as the object's standard electrode potential. The difference between the electrode potentials of the cathode and anode determines the voltage of the entire cell. The anode and cathode are separated by the electrolyte, which is a liquid or gel that conducts electricity. When the anode and cathode are then connected to each other through a wire, the anode undergoes a chemical reaction with the electrolyte in which it loses electrons, creating cations, or positive ions—a process called oxidation. The electrons and cations meet at the cathode where they undergo a chemical reaction called reduction. Together the entire process is known as a reduction-oxidation, or redox, reaction. The electrons travel through the wire from anode to cathode because they are at a higher energy in the anode than in the cathode. When electrons flow through a device such as a light bulb, the battery's energy is used to do work. The chemical reactions in the battery can last for some time, but not forever. Eventually they deplete or corrode the anode and cathode, leaving insufficient material to keep the reactions going.
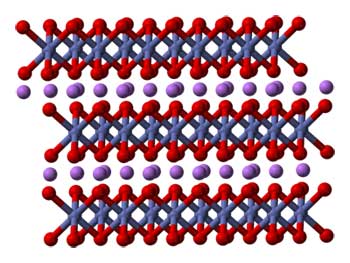
Lithium cobalt oxide consists of layers of lithium (show here as purple spheres) that lie between slabs formed by cobalt and oxygen atoms (shown here as connected red and blue spheres).
In a lithium-ion battery, the lithium ion is the cation that travels from anode to cathode. Lithium (Li) is easily ionized to form Li+ plus one electron. The electrolyte is typically a combination of lithium salts, such as LiPF6, LiBF4, or LiClO4, in an organic solvent, such as ether. Graphite (carbon) is most commonly used for the anode, and lithium cobalt oxide (LiCoO2) is the most common cathode material. This combination gives an overall voltage of 3.6 Volts (V), more than twice that of a standard AA alkaline battery. This gives lithium-ion batteries a much better energy per volume ratio—or energy density—than an ordinary alkaline battery or other common rechargeable battery such as a nickel-metal hydride. This is in part because lithium is the third-smallest element after hydrogen and helium, and thus a lithium ion can carry a positive charge in a very small amount of space. It is important to keep in mind, however, that even lithium-ion batteries are many times less energy dense than substances like motor fuel or food, which store energy in chemical bonds. Increasing the amount of energy that can be packed into a given volume of battery is one of the major challenges facing battery-makers today.
Lithium-ion batteries, unlike standard AA and AAA alkaline batteries, can be recharged by running the anode and cathode reactions in reverse. Typically this is done by a charger that is plugged into a powerful electricity source such as a wall socket or a car cigarette lighter. The ability to be recharged many times over without much loss of capacity is another major advantage of the lithium-ion battery. Imagine if you had to buy a new battery for your cell phone every few days!
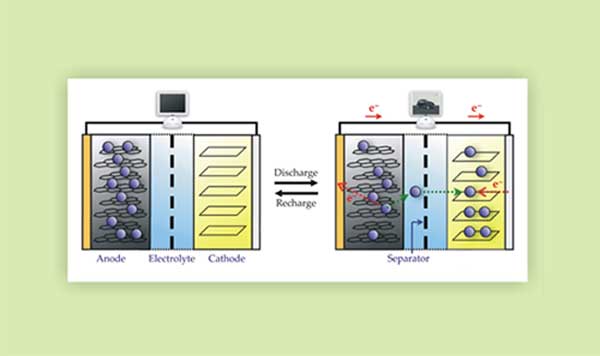
Charging and discharging. Reprinted with permission from Figure 2 from: "Batteries and electrochemical capacitors," by Abruna, Kiya, and Henderson, Physics Today, December 2008. Copyright 2008, American Institute of Physics.
Despite all these advantages, lithium-ion batteries are not perfect. You may have noticed that the amount of charge your cell phone and laptop batteries can hold decreases after a few years. Lithium-ion batteries develop increased internal resistance over time, which decreases their ability to deliver current. In addition, lithium-ion batteries are vulnerable to a number of potential problems, including overheating at the anode (possibly compounded by heat from the device the battery is powering), and oxygen production due to overcharging at the cathode. Put those two problems together and you have good conditions for a fire—exactly what happened to a few unlucky laptop owners.
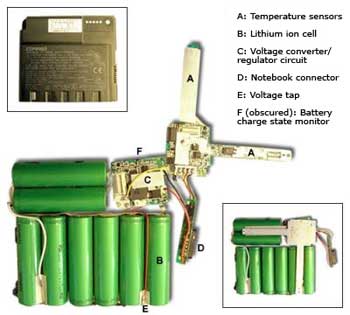
An image showing the inside of a lithium-ion battery pack, with protective devices. Courtesy of ZDNet UK.
Today, lithium-ion batteries are manufactured with protections to limit the charging voltage and to shut off the battery if the temperature becomes too high. Other safeguards allow for venting in the case of buildup of pressure and prevent too-deep discharge, after which the battery cannot be recharged. This protective circuitry does make the battery safe, but it also reduces the fraction of the battery that is used to store energy, and also slowly drains the battery even when the device is off. A number of research groups are in the midst of improving these and other aspects of the lithium-ion battery, and the future looks bright for this hard-working battery to appear in more and more devices, including the electric cars we hear so much about these days.
Research
Much of the recent efforts to improve lithium-ion batteries have focused on developing anode or cathode materials that can hold more charge in a given volume, leading to higher energy densities. Numerous research groups are focusing on replacing the graphite anode with silicon, which could potentially store up to ten times the current capacity. The downside is that silicon films tend to expand when absorbing lithium ions during charging, and shrink again when releasing lithium ions during discharge, leading to pulverization and fracturing of the anode and a short life for the battery. Recently a group led by Yi Cui of Stanford University has used silicon nanowires to create an anode that does not have this weakness. Shown in Figure 3 are images of these nanowires with and without lithium ions, taken using a scanning electron microscope (SEM).
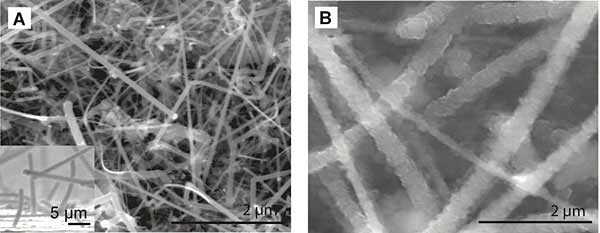
Figure 3: Morphology and electronic changes in Si NWs from reaction with Li. From “High-performance lithium battery anodes using silicon nanowires.” Chan et al. Nature Nanotechnolog, 3, 31 - 35 (2008).
Another idea that has attracted a considerable amount of attention is using lithium iron phosphate (LiFePO4) for the cathode. While it has a slightly lower capacity and significantly lower conductivity compared to lithium cobalt oxide, iron phosphate is both cheaper and less chemically reactive. Yet-Ming Chiang and his colleagues at the Massachusetts Institute of Technology (MIT) have been working to change that. In 2002 they showed that by “doping” (adding impurities to) the iron phosphate, they could achieve much higher conductivities than what had previously been thought possible. And in 2004, Chiang’s team was able to use very small (less than 100 nanometers) iron phosphate particles to improve the cathode’s capacity and conductivity.
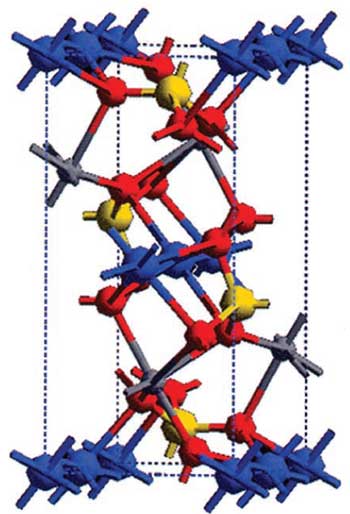
A ball-and-stick model of lithium iron phosphate, where the lithium atoms are blue, the iron atoms are grey, the phosphorus atoms are yellow, and the oxygen atoms are red. From “Electronically conductive phospho-olivines as lithium storage electrodes.” S Cung, J. Bloking and Y. Chiang. Nature Material, Volume 1, October 2002.
Chiang has also been involved in research on advanced assembly techniques. A team of researchers recently used viruses to assemble lithium-ion battery cathodes out of very thin gold and cobalt-oxide wires. Viruses and other biological systems are able to recognize molecules and to assemble themselves into organized patterns, which make them ideal for microscopic battery engineering. As with the silicon anodes described above, these new cathodes take advantage of nanowires’ large surface area, which provides a greater capacity for charged particles.
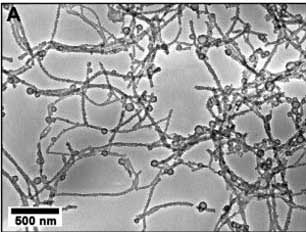
Tunneling Electron Microscope (TEM) image of virus-templated Co3O4 nanowires. “Virus-Enabled Synthesis and Assembly of Nanowires for Lithium Ion Battery Electrodes.” Nam et al, Science, 12 May 2006 Vol 312 p 886.
Other research groups are focusing on novel electrolyte materials. As mentioned earlier, today’s lithium-ion batteries lose capacity over time, largely due to chemical reactions between the electrolytes and electrodes. Mohit Singh of the startup company SEEO is developing a novel electrolyte based on polymers, which are molecules made from long chains of repeating structural units. Singh combined a structurally stable polymer with one that is good at conducting ions to create an electrolyte layer that is both thinner and less chemically reactive than those in use today. Hiroyuki Nishide of Waseda University in Tokyo is developing a totally organic, flexible battery with electrodes made of chains of organic molecules instead of metals. This could avoid problems associated with certain metals, including limited availability and waste disposal. Compared to today’s lithium-ion batteries, Nishide’s offer the potential for faster charging and discharging and longer life, in exchange for, at the moment at least, lower charge densities.
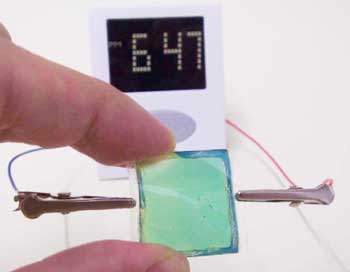
Photograph of Nishide’s flexible polymer battery. From Takeo Suga, Hiroki Ohshiro, Shuhei Sugita, Kenichi Oyaizu, and Hiroyuki Nishide, Adv. Mater. in press (adma200803073).
Schematic showing charging and discharging reactions. From Takeo Suga, Hiroki Ohshiro, Shuhei Sugita, Kenichi Oyaizu, and Hiroyuki Nishide, Adv. Mater. in press (adma200803073).
Whatever the materials of choice for electrodes and electrolytes turn out to be, one thing is clear: for the energy efficient future we all dream of, the batteries of the future, like so many promising technologies, will depend on nanoscale engineering techniques that are still being invented.
Links
HowStuffWorks
How Lithium-Ion Batteries Work
Battery University
A great general-interest website about batteries.
Science@Berkeley Lab
Batteries of the Future II
YouTube
How it’s made: Lithium-ion batteries
Tech-On
Li-Ion Rechargeable Batteries Made Safer
Science Daily
New Nanowire Battery Holds 10 Times The Charge Of Existing Ones
Technology Review
Higher-Capacity Lithium-Ion Batteries
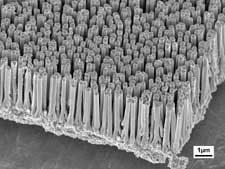
A forest of copper rods about 100 nanometers in diameter create much more surface area for high-capacity battery electrodes. Originally published in "High rate capabilities Fe3O4-based Cu nano-architectured electrodes for Lithium-ion battery applications"
P.L. Taberna, S. Mitra, P. Poizot, P. Simon* and J.M. Tarascon, Nature Materials, 5 (2006) 567-573