Plasma Power
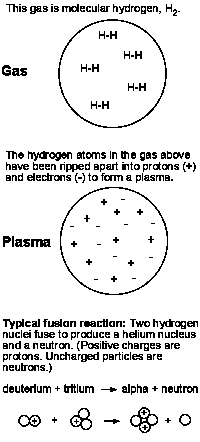
Everyday objects can be classified into solids, liquids, and gases. However, the matter in a lightning bolt, a flame, and the Aurora Borealis are something quite different. Each of these is a plasma, an ionized gas. In a plasma the electrons are ripped from atoms to produce freely-moving ions. Since ions and electrons are charged, they respond to electric and magnetic forces and interact with each other through these forces as well.
Beyond Earth, plasmas are certainly abundant. About 99% of the visible universe is plasma, including most of the matter in stars and the region of space around Earth. This plasma near Earth is controlled by Earth’s magnetic field, or magnetosphere, This magnetic shield is particularly important since it helps insulate Earth from the solar wind, the stream of protons and electrons that flows out into space from the sun.
The inside of a star is an extremely hot, dense plasma. The hydrogen nuclei in this plasma fuel the stellar fusion processes that provide the star’s energy. Harnessing this same process here on Earth would meet our energy needs indefinitely. The raw materials, two isotopes of hydrogen, are readily available, and the by-product, helium, is an inert gas. The great challenge is sustaining here on Earth the high temperatures found at the centers of stars. We are not quite there yet, but fusion researchers have made steady progress over the last few decades.
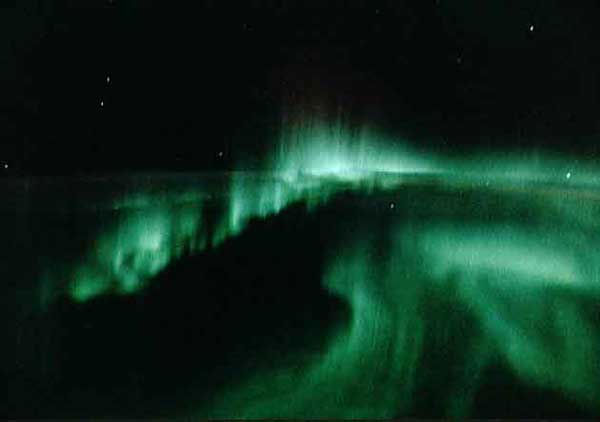
Plasma Power: Fusion Power
As the energy demands of industrialized societies mount, and the limits and risks of conventional energy sources become clear, fusion power is an increasingly attractive alternative. Yet after four decades of research, a working fusion reactor is still at least decades away.
Fusion is difficult to sustain in the lab. Since hydrogen nuclei are charged particles, they experience a strong electrostatic repulsion, which increases rapidly as the nuclei approach each other. For fusion to occur, the nuclei must hit each other at high speed, which requires a temperature of 100 million degrees. At this temperature, any contact with the container would vaporize it, so the nuclei, which are part of a plasma, must be somehow confined.
One approach is to apply strong magnetic fields to keep the charged particles of the plasma off the container walls. The most common containers are shaped like donuts to provide space for the plasma particles, electrons and hydrogen nuclei, to circulate. An electric current induced in the plasma creates a magnetic field that helps confine the plasma.
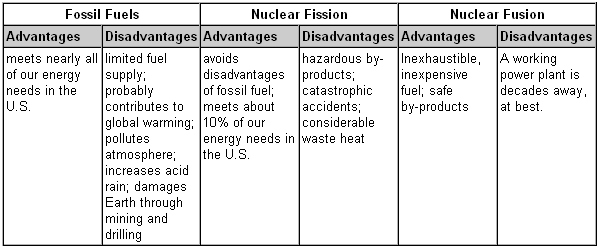
Plasma Power: Plasma Machines
The first photo shows the Tokamak Fusion Test Reactor (TFTR), a large fusion device operated at the Princeton Plasma Physics Laboratory (PPPL) during the 1980s and 1990s. The inside of TFTR was shaped like a donut (a torus). Moving in a circular path around the center of the torus, the plasma reached temperatures of more than 500 million degrees, well beyond the 100 million needed for practical fusion. Ideally, commercial fusion reactors will operate continuously, but TFTR was designed to operate only in bursts. In one of these bursts, its power output exceeded 10 million watts, enough to power more than 3,000 homes.
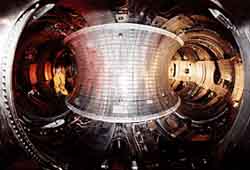
Photo courtesy of Princeton Plasma Physics Laboratory
With the TFTR's research program complete, the PPPL physicists and engineers have built a smaller, nearly spherical device, the National Spherical Torus Experiment, (NSTX), shown in the second photo. Theorists believe that the spherical torus geometry will facilitate plasma confinement and eventually provide a more efficient method of sustaining fusion reactions.
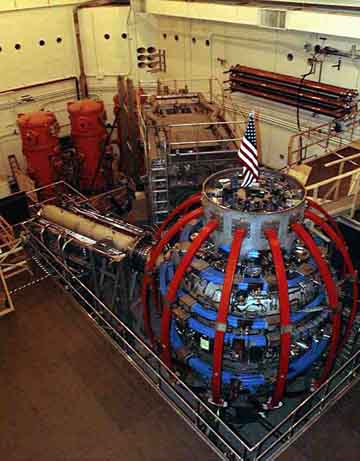
Photo courtesy of Princeton Plasma Physics Laboratory
Links
Princeton Plasma Physics Laboratory
- Interactive Plasma Physics Experience (IPPEX)
- Fusion basics
- Fusion chart
- Fusion resources for students and teachers
Plasmas International
NASA
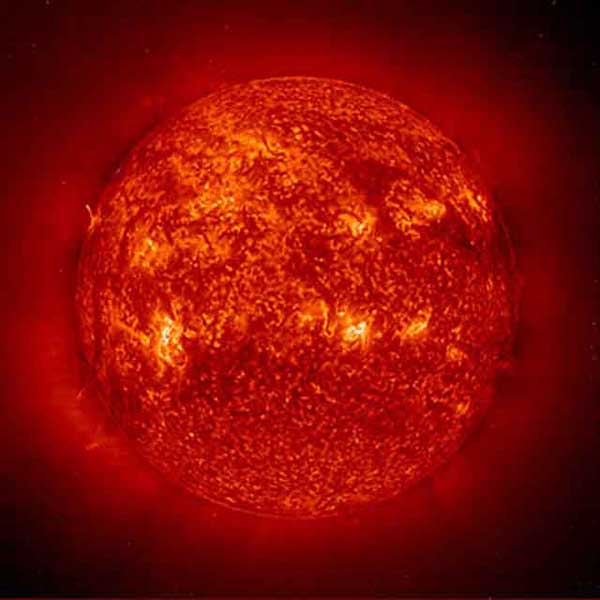
Solar atmosphere as viewed by the Extreme ultraviolet Imaging Telescope