You are My Shining Star
About Supernovae
To a star, size matters. The more massive the star, the higher the pressure and temperature in its core, the brighter it shines, and the sooner it exhausts the hydrogen fuel supply for its fusion reactions. Once the star runs out of hydrogen, its fusion reactions can no longer balance the tremendous inward pull of gravitation, so the star collapses and blows off its outer layers of hydrogen.
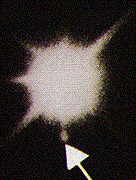
This photograph shows the diffraction of red laser light as it passes through slits of different widths. Notice that the narrower the slit, the wider the diffraction pattern. (photo credit: Jaime Lush, Harvard University Science Center)
What happens next depends on the star’s mass. For small stars, gravity crushes the atoms out of existence, leaving a core of nuclei and electrons--a white dwarf (see image). The core stabilizes at this size due to the distribution of energy in the electrons. Just as with the electrons filling up atomic orbitals, no two electrons in the star’s core can occupy the same quantum state (the Pauli exclusion principle). Many of the electrons in the star are, therefore, forced to occupy high energy states. Their large kinetic energy generates a pressure that is able to resist the force of gravity and stabilize the core of the star at about the size of Earth. The density of a white dwarf is about 109 kg/m3 (106 g/cm3, a million times the density of water).
For larger stars, greater than eight solar masses, the electron pressure is overwhelmed by the inward force of gravity, and the core collapses and then rebounds, resulting in a catastrophic supernova explosion (see image). In the residue left behind, gravity squeezes the protons and electrons together to form neutrons, producing a core whose density is about the same as the atomic nucleus, 1017 kg/m3 (1014 g/cm3, 100 trillion times the density of water). The supernova remnant—what remains of the core—is called a neutron star.
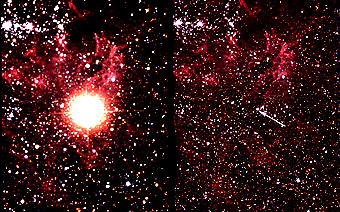
This drawing shows a metamaterial, composed of an array of wires and split metal rings. When illuminated by radiation with a wavelength much longer in the size of the wires and rings, this material has a negative index of refraction. (image courtesy of NASA)
There is an alternate path to a supernova explosion. If a white dwarf resides in a tightly-bound double star system, the dwarf star can accrete matter from its companion, and when the dwarf's mass reaches 1.4 solar masses, a supernova explosion is triggered through fusion reactions. The white dwarf is very dense, so a relatively small solar mass—much less than eight solar masses—is required to trigger this kind of supernova. Both types of supernova formation, a large star or an accreting white dwarf, are quite rare—only a few per century in the Milky Way.
For very large stars, greater than 10 solar masses, the theorists disagree. Some say that the star will undergo such an intense supernova explosion that nothing will remain of its core. Others claim that the star can suffer a succession of "burps", mini-supernovas that blow off some of the star’s outer mass but leave the rest of the star intact and able to explode again. Alternatively, a very large star might produce such energetic gamma rays that photons would convert to electron-positron pairs. Since these pairs produce very little outward pressure, the star would collapse and explode. Since the density of these electron-positron pairs is so much lower than the density of a white dwarf, the electron pressure that stabilizes a white dwarf cannot support the very large star.
As for black holes, they typically are believed to form from large stars—greater than eight to ten solar masses—and the larger the star, the better the odds it will die as a black hole. But beyond the star's mass, its spin is an important variable. Rapid rotation in the core can limit its collapse and rebound, thereby preventing the formation of a supernova, and instead the star collapses right out of existence as a black hole.
Research
In September of 2006, astronomers observed the brightest supernova ever, by far. The image shows SN2006gy, which outshines its own galaxy with an intrinsic brightness 100 times that of a typical supernova. Moreover, SN2006gy’s brightness changes far more slowly than a typical supernova—70 days passed from the first observation of the explosion to maximum brightness, and eight months later it was still brighter than a typical supernova (see light curves). From this brightness, its mass was estimated to be about 150 times the mass of the Sun.
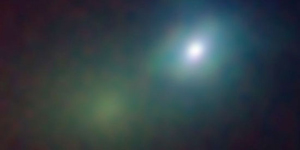
This is an infrared image of SN2006gy and the nucleus of its galaxy. For comparison, a typical supernova is about as bright as its galaxy. (image credit: Lick/UC Berkeley/J. Bloom & C. Hansen)
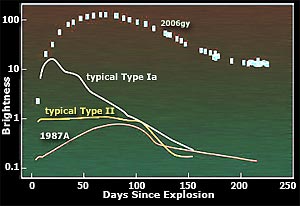
These graphs show light curves for various supernovas. Note how slowly SN2006gy's curve changes compared to the others, and how much brighter it was. (image credit: Nathan Smith, UC Berkeley)
![This Hubble image shows lEta Carinae, an extremely large star in the Milky Way that survived an outburst about 150 years ago. This star is a potential supernova. (image credit: J. Morse [U. Colorado], K. Davidson [U. Minnesota], STScI, and NASA) This Hubble image shows lEta Carinae, an extremely large star in the Milky Way that survived an outburst about 150 years ago. This star is a potential supernova. (image credit: J. Morse [U. Colorado], K. Davidson [U. Minnesota], STScI, and NASA)](/explore/action/images/supernova-img5.jpg)
This Hubble image shows lEta Carinae, an extremely large star in the Milky Way that survived an outburst about 150 years ago. This star is a potential supernova.(image credit: J. Morse [U. Colorado], K. Davidson [U. Minnesota], STScI, and NASA)
Some astrophysicists hypothesized that this star was a white dwarf in a binary system that was accreting mass from the companion. In this model, the white dwarf ultimately would explode into the hydrogen it was accreting and produce an intense x-ray flux. To test this idea, the Chandra telescope observed SN2006gy in x-rays, and the results were too small by a factor of about a million to fit the accreting white dwarf model.
Although we on Earth reside at a safe distance—about 24 million light years—from SN2006gy, we have an ominous-looking neighbor right here in our own Milky Way, a mere 2500 light-years away (see image). This star, Eta Carinae, has a mass of between 100 to 120 solar masses, so it's one of the largest stars in our galaxy. It also has a lurid history (see table below), including a "false supernova" explosion in 1843, which split it into two spherical lobes separated by a thin disk, as shown in the image.
With its great mass and history of instability, Eta Carinae is certainly a potential supernova. If it were to explode, it could emit powerful jets of gamma radiation, which would threaten the Southern Hemisphere, but fortunately these jets are narrow and well separated. In any case, astronomers plan to keep a close watch on this star in the future.
Year | Magnitude (the more negative the magnitude, the brighter the star) |
1677 | 4 |
1730 | 2 |
1782 | 4 |
1827 | 1 |
1843 | -1 (it looked like a bright star in the night sky) |
1868 | Invisible |
1953 | 7 |
1990 | 6 |
1998 | Suddenly became twice as bright |
Links
Solstation
Students for the Exploration and Development of Space
HubbleSite
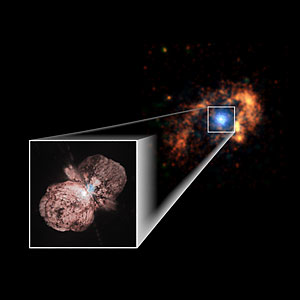
This image shows Eta Carinae, in both the visible—inset and blue, and in radio—the orange nebula. (image credit: Chandra Science Center and NASA)
Wikipedia
NASA/Goddard
NASA