Optical Tweezers
Unwind a chromosome to see how it’s put together? Sort cells with a light beam? Make a model of a molecular motor? All these and more—welcome to the world of optical tweezers, where cells and even individual molecules are manipulated with laser light.
How does it work? When light passes through an object, the light refracts, that is, it changes direction. It does this as it enters the object, as it passes from one substance to another inside, and as it exits. Photons, the quanta of light, carry momentum, and the light’s momentum is changed by being bent as it passes through the object. To conserve the total momentum, the object itself acquires momentum equal to that lost by the photons, and this momentum can be used to move the object into a trap in the optical system.
To make a trap, a laser beam is set up with an intensity that diminishes moving out from the center of the beam, as shown in the diagram. Lenses bring the beam to a focus, the point of maximum light intensity.
The next diagram shows a translucent sphere placed in the laser beam between the lens and the focus. The solid and dotted line show the paths of a typical pair of photons that pass through the object. Because the two photons wind up going more sideways than initially, they lose momentum in the downward direction. To conserve momentum, the object acquires momentum in the downward direction, towards the focus.
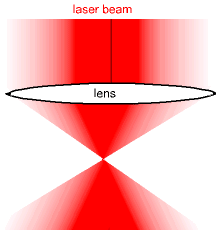
Beam Intensity— A schematic drawing of the laser beam brought to a focus in optical tweezers. The beam intensity decreases going out from the beam axis.
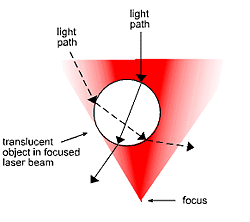
Trapped Sphere — Two light paths in the optical tweezer laser beam passing through a translucent object. Note that the path that originates closer to the center of the beam will have the greater number of photons per second. The changes in momentum of photons on these paths gives the sphere momentum toward the beamaxis and also toward the focus .
Research
Physicists use optical tweezers to investigate how the genetic information in chromosomal DNA is “read” during cell reproduction. The base sequences that carry the genetic code lie between the two sugar-phosphate backbones of the DNA molecule, and these bases must be exposed to replicate the DNA. In the chromosome, though, the DNA is wound around and around proteins, which in higher organisms are called histones. This wrapping is functional, since it enables the long DNA molecules to fit into the cell, but the DNA must be unwrapped before it is copied. In life, the enzyme DNA polymerase makes the copy, and associated proteins unwrap the DNA biochemically from the histone.
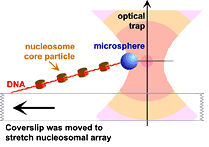
Optical tweezers hold up one end of the DNA/histone complex, while the other end is fastened to a microscope slide. The slide is slowly moved to unwrap the DNA from the histone. (drawing courtesy of Michele Wang/Cornell University)
A model of how DNA unwraps. Note that initially several turns of the DNA are wrapped around the histone, and that only after complete unwrapping does the histone break off. (image © Cornell University, used with permission).
A group of biophysicists at Cornell and the University of Massachusetts investigated the beginning of this process by unwinding DNA from the histone mechanically. They attached one end of a DNA strand to a microscope slide and the other to a tiny transparent sphere. Then they pulled on the sphere with optical tweezers, like a mini tractor beam, to unwind the DNA, as shown in the diagram.
The group found that the DNA unwound in distinct stages, with increasing force required at each stage (see second diagram). Remarkably, even with 156 bases unwound from the histone, releasing the force enabled the DNA to spontaneously rewrap and return to its original state. These results showed that the enzymes that unwrap the DNA must be “molecular motors,” capable of exerting forces, and some of these have indeed been found.
This work is just one of the many applications of optical tweezers.
Links
University of Chicago
Lawrence Berkeley Laboratory
St. Andrews University
Cornell University

Eight spheres are manipulated into a cubic arrangement with overlapping optical tweezers. When the laser is turned off, the cubes gradually lose their order. (image courtesy of Optics.org)