Nuclear Forensics and Unbaking the Cake
By H. M. Doss
All year long people and objects enter our country. At our nation’s ports, cargo ships from all over the world, carrying goods from granite to rubber duckies, enter the United States. The cargo is unloaded from a ship and onto a truck to be transported to its final destination in the U.S. But how do we know what’s really in each cargo box and if it is safe?
One safety check requires each truck to pass through radiation monitors to see if there is any radioactive substance in the cargo entering the country. A radioactive substance emits ionizing radiation, energetic electromagnetic waves or particles that can knock off parts of atoms or molecules it interacts with. This is concerning because it can damage or destroy living cells. If an alarm is set off, a Customs and Border Patrol officer has the truck go through a second radiation monitor and checks the vehicle with a handheld radio-isotope identification device or RIID. The RIID measures the ionizing radiation. Often the RIID displays on its screen an “unidentified” graph related to the energy of the ionizing radiation emitted by the radioactive material. These handheld devices have a difficult time distinguishing mixtures of radioactive isotopes. At this point a scientist, who understands the energy levels of nuclei and the pattern of energy they emit (gamma ray spectroscopy), is sent the data to analyze and to determine what radioactive substances are present.
Characteristic properties similar to fingerprints
The gamma-ray spectrum of a nucleus is similar to a fingerprint of a human. Each nucleus has distinct energy levels, causing it to be able to absorb and emit specific types of radiation. Visible light and gamma rays are part of the electromagnetic spectrum. When an electron bound to an atom absorbs just the right amount of energy, it will go to an allowed higher energy level. When an atom’s electron makes a transition to an allowed lower energy level, it emits electromagnetic radiation, which may be in the microwave, infrared, visible, X-ray, or other portions of the electromagnetic spectrum. These energy levels are determined by quantum mechanics and are similar to steps rather than a ramp. Just as you cannot place your foot down between two steps, a bound electron cannot be in any location - it must be in an allowed energy level. Within the nucleus there are also allowed energy levels that the protons and neutrons must be in. Gamma rays are produced by transitions between energy levels within the nucleus.
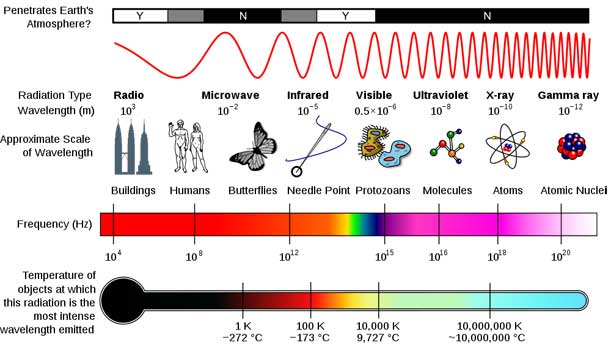
Because every element has its own allowed electron energy levels, the spectrum of light produced by each element is unique. The spectrum serves as a characteristic property (something like a fingerprint) for each element, which allows scientists to identify the elements present in a sample. An isotope of an element has the same number of protons but a different number of neutrons in the nucleus of the atom. Every nuclei, including each isotope of each element, has a characteristic or unique gamma ray spectrum.
![]() a |
![]() b |
Gamma ray spectra for (a) uranium ore and (b) cobalt-60. The two peaks for Cobalt-60 occur at 1.17 MeV and 1.33 MeV. |
Common granite contains veins of uranium ore, and may set off radiation detectors as it comes through one of the ports of entry. Bananas and kitty litter naturally contain the radioactive isotope potassium-40 and large quantities of these items may set off the radiation detectors. Sometimes large amounts of cobalt-60 are found in metal products because not enough care was taken in determining which metals in a scrap yard were being melted together. For example, medical equipment often contains cobalt-60 and if this metal gets mixed in with other common metals, then we may end up with radioactive rebar (used in buildings) or table legs. This was the case for metal produced at the Ciudad Juarez, Mexico site in 1983 that prompted the installation of radiation detectors at border crossings.1 Scientists viewing gamma ray spectra can determine if there are improper levels of radioactive substances in products we import.
“Unbaking the Cake”
But what if highly enriched uranium or plutonium were to enter the country? If that were to occur, the substance would be transferred to a national lab for analysis. It is in these labs that nuclear forensic scientists “unbake the cake” by figuring out what materials they have, how much of each material is present, how much of each material was originally used in the recipe, where it was processed, how it was processed, when it was processed, and any clues that might help law enforcement authorities identify the people involved. Experts in radiochemistry, nuclear engineering, nuclear physics, geochemistry, and/or geophysics, and other science fields, work together applying physics concepts to determine the answers they need.
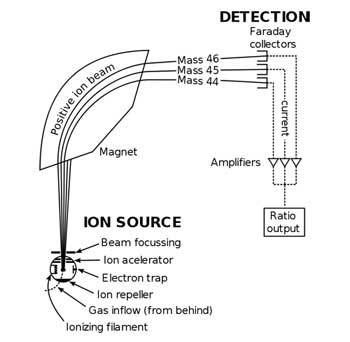
Diagram of a typical mass spectrometer.
By analyzing the gamma ray spectrum, scientists can tell which isotopes are present. They double-check this with a mass spectrometer, which also gives them information about the ratio of isotopes present. When using a mass spectrometer, scientists take a sample of the radioactive substance and dissolve it into solution, or ablate it (a layer of substance is removed from the surface) using a laser. The sample is blown into a plasma (a mixture of ionized atoms and unbound electrons) that is hotter than the surface of the Sun! While in the plasma, the sample itself becomes part of the plasma. It is completely ionized meaning all the electrons are stripped off of the atoms. What remains are nuclei of various elements and isotopes, all positively charged, and electrons, not bound to an atom. The ionized sample is then accelerated in a magnetic field, which makes the charged particles turn. Positive charges turn one way and negative charges turn the other. This property is used to remove the electrons from the sample. How sharply these charged particles turn depend on their mass to charge ratio. The smaller the mass to charge ratio of the isotope, the sharper the turn that it makes. Collecting the positive ions after they travel a little further along their new paths allows scientists to separate out all the different isotopes by seeing where they hit a detector. They can determine how many of the isotopes hit the detector by the current produced by the detectors. Current is just the amount of charge that flows past a surface per second. From these measurements obtained using a mass spectrometer, scientists can determine all the isotopes present and the ratio of these isotopes. This information provides scientists with all the ingredients used as well as the ratio of ingredients.
Scientists use the mass spectrometry data to gain insight about when the last time the uranium was enriched (or purified) or when the plutonium was produced. They do this by looking at the ratio of different isotopes. Radioactive nuclei decay over a certain time period. Their half-life is determined by how much time goes by for half of the original nuclei to decay to a new, daughter-nuclei. By looking at all the nuclei, scientists can easily determine to within a few years (or less) when the material was last enriched or produced. This information provides clues as to which country processed it, since countries often have different ratios of ingredients. Using relative concentrations of parent to daughter isotopes to determine the time since last purification is known as “radiochronometry.” To learn more about decay times and play with some fun computer simulations, click here.
Each country also has their own technique for processing uranium, which may produce different grain sizes and structures in the final material product. Nuclear forensic scientists use electron microscopes, and scanning tunneling microscopes as part of their toolbox to view the grain structure of the material. This is similar to a cake’s texture. Is it dense, moist, or fluffy? What size are the air pockets in it? Answers to these questions give clues to how the cake was produced.
![]() a |
![]() b |
Schematic diagrams of (a) an electron microscope, (b) a scanning tunneling microscope. |
Using the various microscopes available scientists can also find out information about where any part of the containers were made that the radioactive substance was in. Sometimes these nuclear forensic scientists get lucky and find a bit of pollen embedded in glass that helps them determine the country a container was made in. Determining compositions of containers, including the ratio of the elements found, provide clues to where those containers were created because each place on Earth has a different ratio of elements in its soil. All this information helps law enforcement officers track down where and who was involved in potential illegal production and/or transport of uranium or plutonium.
The International Atomic Energy Agency2 (IAEA) keeps records of all legally processed uranium and the date of enrichment.
Research
One active area of research is in design of ionizing radiation detectors.3,4 New detectors are being developed that utilize cosmic background radiation to detect radioactive substances.5
The Glenn T. Seaborg Institute6 has current research projects involved with finding and studying new elements, analyzing chemical and isotopic signatures at the nanoscale for both biological and nuclear forensic studies, considering how nuclear waste and underground test sites may release radioactive nuclei over time using environmental radiochemistry, and studying how particles of plutonium are transported in water and ground water.
Links and References
1. http://en.wikipedia.org/wiki/List_of_civilian_radiation_accidents
2. International Atomic Energy Agency (IAEA)
3. Physics Today, September 2010
http://blogs.physicstoday.org/newspicks/2010/09/new-advance-in-radiation-detec.html
4. Long, J, The Importance of Mobile Radiation Detection for Homeland Security, EHS Today
http://ehstoday.com/industrial_hygiene/instrumentation/importance-mobile-radiation-detection-homeland-security-0510/
5. Wolverton, M., A Sensor that Tracks Cosmic Particles that couls Spot Hidden Nuclear Threats Before They Cross Our Borders, Popular Science, September, 2010
http://www.popsci.com/technology/article/2010-07/drive-thru-detection
6. Glenn T. Seaborg Institute, facilitates research collaborations between LLNL and the academic community in radiochemistry and nuclear forensics through world-class research in the areas of:
- Nuclear forensics and attribution
- Heavy element discovery
- Radiochemistry for National Ignition Facility (NIF) diagnostics
- Environmental radiochemistry
https://www-pls.llnl.gov/?url=about_pls-centers_and_institutes-glenn_t_seaborg_institute
7. Moody, K.J., Hutcheon, I.D., Grant, P.M., Nuclear Forensics Analysis, Taylor & Francis: Boca Raton, FL, 2005.
8. Department of Homeland Security
http://www.dhs.gov/xabout/careers/content_multi_image_0011.shtm