Quark-Gluon Plasma
A millionth of a second after the Big Bang, the universe was an incredibly dense plasma, so hot that no nuclei nor even nuclear particles could exist. The plasma consisted of quarks, the particles that compose nucleons and some other elementary particles, and gluons, the massless particles that “carry” the force between quarks (See Nuclei Knockdown). Gluons are the particles that quarks exchange as they interact, or, in the language of modern physics, gluons “mediate” the strong force between quarks. Since quarks make up protons and neutrons, this leads to the force that holds protons and neutrons together in a nucleus.
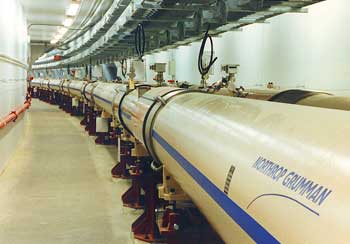
The twin curved tubes at RHIC that contain the counterrotating gold beams, which can be brought together to collide at six instrumented areas around the ring. (image courtesy of Brookhaven National Laboratory)
At RHIC, these coils of wire use radiofrequency radiation to further accelerate the gold ions. (image courtesy of Brookhaven National Laboratory)
The spray of thousands of particles produced from collisions of colliding gold nuclei. (image courtesy of Brookhaven National Laboratory)
A plasma (see Plasma Power) is an ionized gas, like the matter in a spark or a lightning bolt. But unlike these plasmas, as its name implies, the abovementioned quark-gluon plasma is made of exotic particles. Moreover, the way that quarks and gluons interact is unlike any other particles—at low temperatures and densities, they attract each other more strongly as they are separated, which explains why free quarks are not found in nature.
Nuclear and particle physicists have long dreamed of making this exotic plasma in a laboratory and investigating it experimentally. Because of the enormous energies required, the lab would have to be a particle accelerator, with the plasma produced as the result of a collision between particles accelerated towards each other in counterrotating beams, rather than by particles slamming into a fixed target. In the case of a fixed target, not all of the incoming particle energy is available for the reaction, since much of it must go into the kinetic energy of the products in order to conserve momentum. Even with colliding nuclei, much of the energy winds up with large fragments of the nuclei and thus is unavailable to produce the plasma. The nuclei are chosen for large atomic weight to make the collision energy as large as possible.
A team at the Center for Nuclear Research (CERN) in Geneva made a preliminary attempt with a beam of lead nuclei and fixed targets of various materials, but the results, although tantalizing, were not conclusive.
The Relativistic Heavy Ion Collider (RHIC—see Nuclei Knockdown) at Brookhaven National Laboratory was built expressly to produce the quark-gluon plasma. In the RHIC 2.4-mile-long ring, fully-ionized gold ions go in both directions at once and can meet at six places around the ring for collisions (see first link).
Earlier RHIC results suggested that when gold nuclei collide head-on, their kinetic energy dissociates many nucleons and forms the hot, dense plasma of quarks and gluons, which must immediately begin to expand and cool. The hot plasma lasts only 10-23 seconds, and only when the plasma cools sufficiently do the quarks and gluons “freeze out,” leaving a spray of thousands of elementary particles (see image) carrying the signature of the hot, dense plasma that led to their production. To show the minute quantity of plasma produced, if RHIC ran for twenty years, it would consume only about one gram of gold.
Research
In the latest RHIC results, four different experiments show evidence of the quark-gluon plasma. Moreover, in RHIC physicists have drawn the stunning conclusion that this plasma has properties of a liquid, rather than the gas that had been predicted, and that this liquid has no viscosity, making it the most perfect ever observed (Viscosity is resistance to flow—materials like honey have high viscosity.).
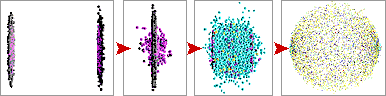
A simulation of a gold-gold collision at RHIC. Due to relativistic effects, the colliding nuclei appear like pancakes as they approach each other. Shortly after they collide, the quark-gluon plasma (purple) forms, but it rapidly cools and condenses into elementary particles (green), which themselves decay into the other particles shown in the last frame on the right. (image courtesy of Brookhaven National Laboratory)
A detailed analysis of the motion of the particles of the plasma—the quarks and gluons—shows that they move together, with their paths coordinated. This tendency for parts of a fluid to flow together, in the same direction, characterizes a zero-viscosity fluid, whereas in a viscous material like honey, parts of the fluid resist flow, so different parts move in very different ways.
Equally striking is the possible connection with string theory (see An Especially Elegant Universe). In string theory, particles can be described as vibration modes of tiny strings, much too small to be detected themselves but, according to string theorists, manifest in elementary particles and gravitation. In fact, string theory was invented to bring together these two areas of research, which so far have been successful only separately. Although an active area of investigation in theoretical physics, string theory has been repeatedly demeaned as incapable of being tested by experiment.
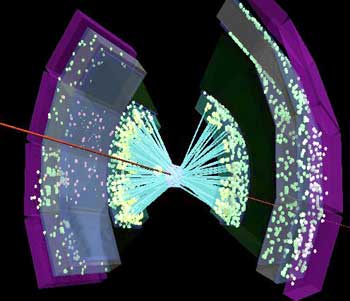
This image shows the products of a RHIC gold-gold collision. The white and yellow dots indicate where particles were detected, and the blue-green lines show calculated particle tracks. (image courtesy of Brookhaven National Laboratory)
It turns out that a string theory set up in a ten-dimensional space can describe mutually interacting quarks, and in fact string theory methods can be applied to predict the measured viscosity of the quark-gluon liquid.
Many physicists are cautious, however, because there is some question that string theory methods should even apply in this case.
Whether or not the RHIC results are explained by string theory, this new evidence for the quark-gluon plasma, with properties of a perfect liquid, is an astonishing result. Much work remains to characterize the plasma and especially its transition to the elementary particles that appear in profusion when the plasma freezes out. This is physics at its most fundamental, investigating both the matter at the beginning of the universe and the force— the “strong interaction”—that holds nuclei and quarks together.
Links
RHIC
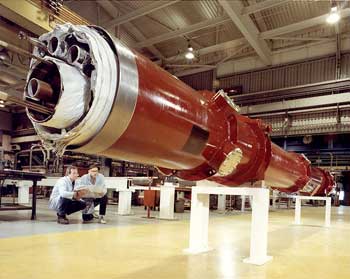
The tubes in the RHIC accelerator are filled with pipes, wiring, and insulation. The magnet wiring, made of niobium titanium, is cooled with liquid helium to about ten degrees above absolute zero so the wiring becomes superconducting, enabling current to flow without electrical resistance. (image courtesy of Brookhaven National Laboratory)