Superconductors
About Superconductivity
How would you like to board a Maglev train and then speed off to your destination at more than 300 miles per hour? The magnets that levitate these trains are an application of superconductivity.
Metals are good conductors of electric current. That is, they have very low electrical resistance, but this resistance is not zero. A voltage difference is still required to generate the current in the metal, and the metal heats up while the current is flowing.
The electrical resistance of an object depends on its temperature and declines slowly as the temperature falls. Early in the last century, however, a Dutch physicist discovered that a sample of mercury, when cooled below a certain temperature close to absolute zero, loses all electrical resistance. When the mercury is in this state, an electric current flows indefinitely, even in the absence of any applied voltage. This effect is called "superconductivity." The table lists the everyday metals that exhibit superconductivity and the temperature below which electrical resistance disappears. These elements require cooling by liquid helium to become superconductors. Such materials are called "low-temperature superconductors."
Much later, in the 1980s, physicists discovered ceramic compounds that exhibit superconductivity at temperatures as high as -145º Celsius. This temperature is high enough that the materials need be cooled only with liquid nitrogen, which is far less expensive to do than with liquid helium.
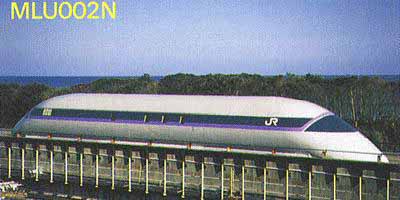
Japanese Maglev train prototype (photo courtesy of Railway Technical Research Institute, Maglev Systems Development Dept.)
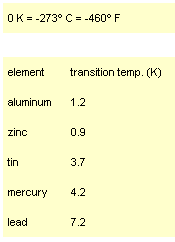
Applications of Superconductivity
The early superconductors were chunks of metal. A breakthrough came in the 1960s with the development of a superconducting wire, an alloy of niobium and titanium. With this wire, engineers could wind electromagnet coils. These superconducting coils permit the construction of extremely powerful electromagnets. As with many engineering advances, there are tradeoffs:
Cost Saving: Since the magnet is operated with the wire at superconducting temperatures, the resistance of the coils is zero and no energy is lost to heating the coils. For superconducting magnets, a small power supply is sufficient to initiate the flow of current.
Cost Increase: Since the coils must be maintained at a low temperature, an expensive liquid helium refrigeration system is required.
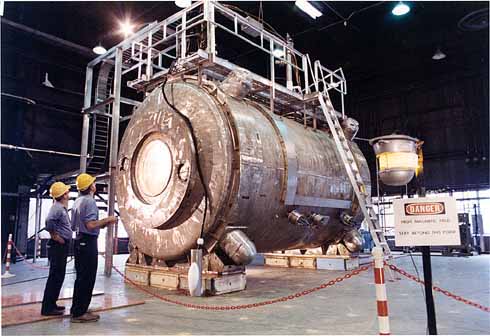
200-ton superconducting magnet built at Argonne National Laboratory as part of a project to investigate power generation from fusion (see Plasma Power in Physics in Action Archives); photo by Dan Giroux, courtesy of Argonne National Laboratory
Maglev Train
A future application is the Maglev train, now under development in Japan. To minimize friction, powerful onboard superconducting magnets support this train above the tracks. The prototype train shown in the photo reached a speed of 411 km/hr (256 mi/hr).
Running alongside the track are walls (see photo) with a continuous series of vertical coils of wire mounted inside. The wire in these coils is not a superconductor. As the train passes each coil, the motion of the superconducting magnet on the train induces a current in these coils, making them electromagnets. The electromagnets on the train and outside produce forces that levitate the train and keep it centered above the track. In addition, a wave of electric current sweeps down these outside coils and propels the train forward (see drawings).
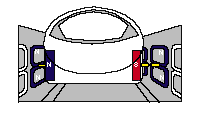
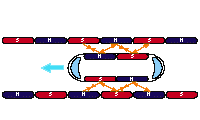
Drawings of Maglev train showing magnets both on-board and built into sidewalls; drawings courtesy of Railway Technical Research Institute Maglev Systems Development Department
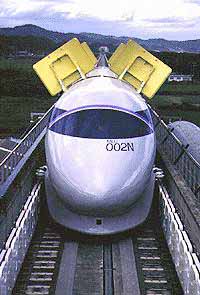
Japanese Maglev prototype MLU0002N with aerodynamic brakes deployed; photo courtesy of Railway Technical Research Institute Maglev Systems Development Department.
MRI Scanners
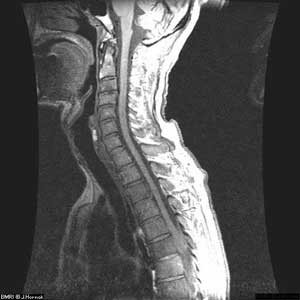
MRI of lower human spine (image used with permission, J.P. Hornak, The Basics of MRI)
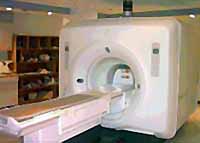
MRI imager (© 2000, Joseph Hornak, The Basics of MRI)
MRI (Magnetic Resonance Image) scans produce detailed images of soft tissues.
The superconducting magnet coils produce a large and uniform magnetic field inside the patient's body. Note the large size of the scanner, which contains the liquid helium refrigeration system.
Particle Accelerators
One important application of superconducting electromagnets is within an area of fundamental physics research-the study of the elementary particles. In collider-style particle accelerators, magnets steer charged particles along counter-circulating paths so the particles can smash into each other at high energy. Modern colliding accelerators are now built using superconducting magnets because the high energies needed by researchers are not attainable without the high magnetic fields created by these magnets. Because superconductors do not have electrical resistance, accelerators built with superconducting magnets are also much less costly to operate. In fact, modern colliders could not be built or operated using conventional (non-superconducting) magnets. Now under construction, the Large Hadron Collider at CERN in Switzerland has a circumference of 27 km (about 16 miles) and requires 8,000 superconducting magnets and 700,000 liters of liquid helium.
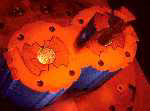
Model of a superconducting magnet for the particle accelerator now under construction at CERN. (photo courtesy of CERN)
The Promise of Superconductivity
Imagine walking into a hardware store to look for wire and the clerk asks if you'd like "regular or superconducting." This ultimate promise of superconductivity-a practical material with no resistance at room temperature-may never be fulfilled, but researchers are hoping to develop a superconductor that will revolutionize our everyday world.
There are two main issues in superconductivity that must be dealt with in order to develop practical technology. First, the material must be abundant and easily manufactured. The so-called "low-temperature superconductors" are primarily niobium compounds- not an abundant substance. The ceramic, high- temperature superconductors are complex mixtures of yttrium, barium, carbon, and oxygen and are difficult to make into wire. Second, the material must exhibit superconductivity at a high enough temperature to limit the cost of cooling the material to its transition temperature. The low-temperature niobium compounds require large amounts of costly liquid helium to maintain superconductivity, but the high-temperature ceramics only require less expensive liquid nitrogen.
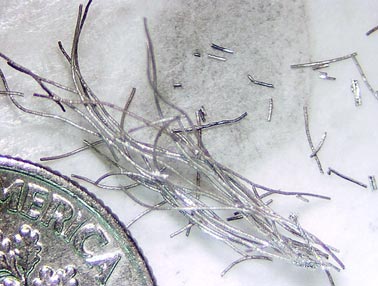
Magnesium diboride wires as they appear after removal from the tantalum tube and part of a U.S. dime for scale. Photo courtesy of Paul Canfield and the U.S. Department of Energy's Ames Laboratory.
In January 2001 a team of Japanese physicists provided an adrenaline shot to superconductor research by announcing that they had observed superconductivity in an abundant magnesium-boron compound, MgB2. While the transition temperature, 40K, of the magnesium diboride is still lower than that of high- temperature ceramic compounds, it is much higher than ever observed in an intermetallic compound and requires only half as much liquid helium. Researchers have already begun to develop techniques for creating magnesium diboride wires, and have shown that the crystal structure of the compound allows it to carry far more electrical current than ceramic superconductors.
Given the fast progress already made with developing this new superconductor, researchers are optimistic that they will be able to tweak the chemical structure to raise the transition temperature to within the reach of liquid nitrogen. But even if this proves impossible, magnesium diboride already promises to surpass the niobium compounds as the low-temperature superconductor of choice.
Links
Argonne National Lab
Department of Energy
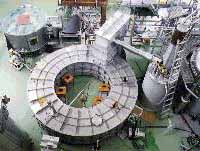
This cylindrical container holds a liquid helium refrigeration system that cools a coil of superconducting wire. The coil generates magnetic fields for a project to investigate power generation from fusion (see Plasma Power in Physics in Action Archives). This coil conducted a current of 21 x 103 Amps.