Puncturing Prey
May 25, 2016
Fangs, claws, tentacles, and sometimes even beaks are dangerous puncture weapons. The tiny trap-jaw ant clamps its jaw shut on prey at speeds nearing 150 mph, and the fangs of the giant king cobra deliver poison that can kill an elephant. Although these animals differ in so many ways—size, habitat, body structure, diet, predators—they both rely on the ability to fracture the tissue of other animals in order to survive.
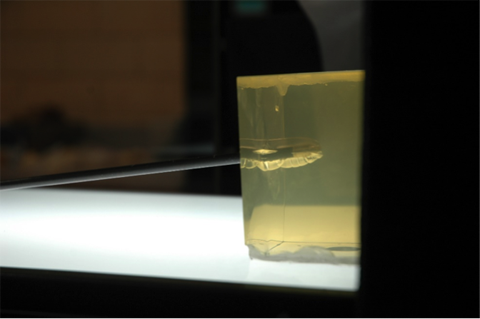
A block of gelatin after being punctured by an arrow for the experiment.
Image Credit: Philip Anderson
Animals commonly subdue prey and defend themselves by puncturing, but we don’t actually know very much about the mechanics behind animal punctures. For example, what is the relationship between the speed of a puncture and the damage it causes? The size of the weapon and its ability to inflict harm? How can we talk about the effectiveness of a jellyfish’s sting versus a snake’s bite versus a woodpecker’s drill? How have these puncturing systems evolved? Are there lessons we can learn from the structure of puncture weapons that could revolutionize our own devices?
An interdisciplinary team of researchers recently started building a framework to answer questions like these with high-speed puncturing experiments, described in the journal Interface Focus. The team includes paleontologist and evolutionary biologist Philip Anderson from the University of Illinois, physics teacher Jeff LaCosse from Charles E. Jordan High School, and mechanical engineer Mark Pankow from North Carolina State University.
In the experiments, the team shot arrows of different weights and at different speeds into blocks of gelatin. With the help of some high-speed video cameras, they explored the relationship between the damage inflicted and the characteristics of the arrow’s motion; in particular, the velocity, energy, and momentum of the arrow.
Taking Aim
Scientists have a fairly good understanding of the science surrounding cutting materials when it is done at a slow and steady rate, but not so much when it comes to animals that puncture at high-speeds—consider that a jellyfish’s hypodermic needle can impact a subject at 85 mph and certain termites can strike at speeds around 125 mph. Clearly the speed of the weapon must impact the amount of damage caused in these cases. In addition, the animals we are talking about don’t inflict harm at a steady rate—the puncture wounds tend to be brief and quick. This isn’t described well by current theory either.
Despite the important differences, slow and steady cutting science still offers a good place to start when it comes to high-speed puncturing. The theory concentrates on how energy is transferred from the cutting tool to the target. When you cut something, you create new surfaces. Think about cutting a cake—a cake cut into pieces will dry out faster because it has much more surface area. The energy you put into cutting goes into creating these new surfaces.
Before taking aim, the team also considered the situation from a ballistics penetration point of view. If you are a fan of CSI shows, you’ve probably seen protagonists fire bullets into blocks of gelatin or dummies that mimic human tissue in order to investigate injury patterns and learn about the conditions under which an injury occurred. Ballistic penetration is a type of high-speed puncture, and the effectiveness of the bullet (or other projectile) is often explored with three variables:
- Velocity, which determines when a projectile will penetrate the material
- Momentum, which relates to the force the target feels on impact
- Energy, which relates to the damage caused by the projectile.
Based on these two areas of study, the researchers decided to explore how velocity, momentum, and energy impact the damage caused by a puncturing weapon.
The puncturing velocity of various animals, given in meters per second.
Image Credit: Graphic by Julie McMahon
Firing
Want to investigate puncturing? Puncture something!
Using a custom crossbow, the team shot arrows at 4-inch blocks of gelatin. They did this 20 times, at various speeds and with arrows of different weights. The whole process was captured by two high-speed video cameras, one focused on the arrow and one on the gelatin. You can check out videos here and here.
Reviewing the footage revealed the following pattern:
- On impact, the arrow entered the gelatin and an area of gelatin near the point of impact was deformed and pulled inward.
- The impact caused stress waves to travel through the block ahead of the arrow.
- As the arrow traveled further into the block, it started to tear the gelatin that was pulled inward, creating new surfaces (as in the cake analogy from earlier).
- The surface of the block began to noticeably deform.
- The arrow stopped and was pushed backward by the energy built up in the gelatin, to the point of being ejected completely about half of the time.
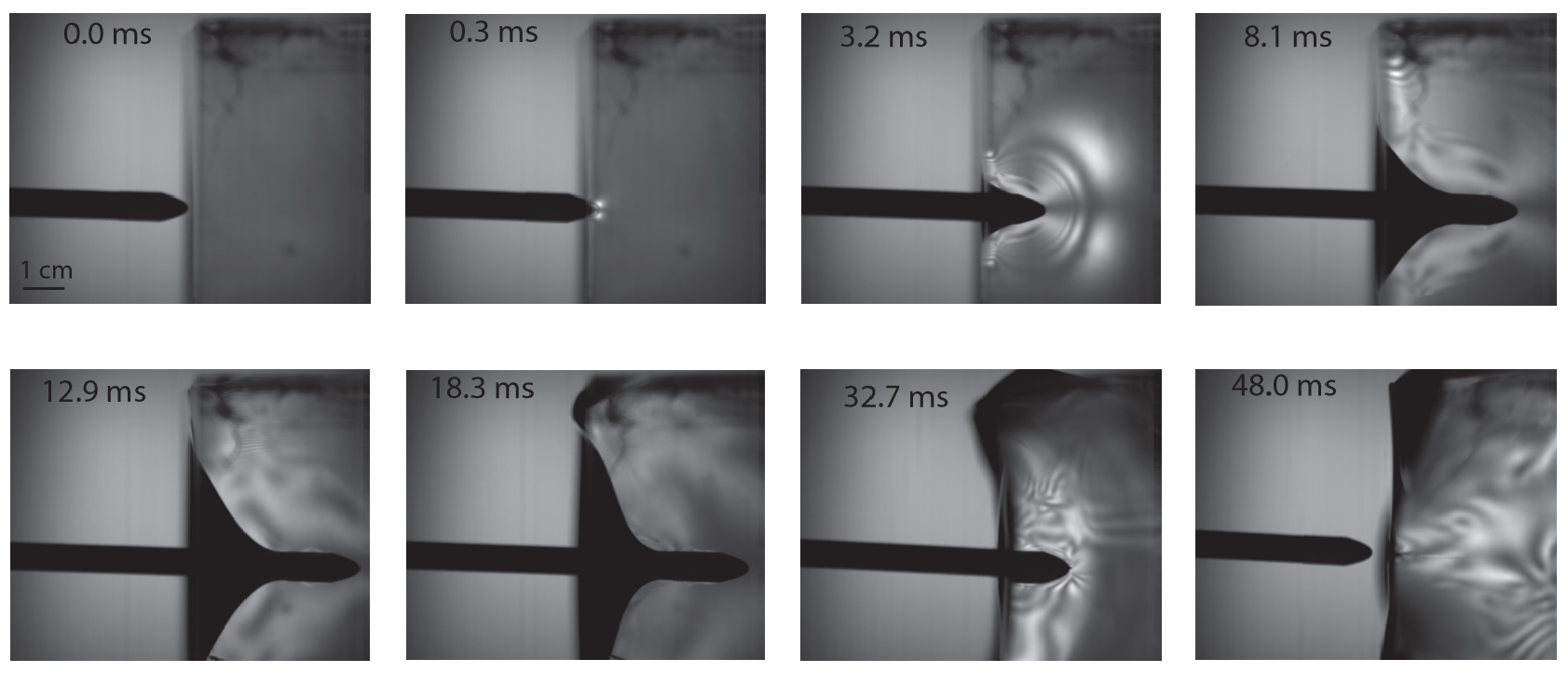
These frames are from a high-speed video of a puncture event, filmed at 20,000 frames per second, with an arrow traveling at 12.2 meters per second (about 27 mph). The gelatin appears as a clear block, while the arrow is backlit and appears black. Stress waves are clearly visible 3.2 ms after impact and the arrow reached its final penetration depth by 12.9 ms. However, the gelatin did not begin to macroscopically deform until 18.3 ms after impact. Finally, the deformation of the gelatin resulted in the arrow being expunged 48 ms after initial impact. (Watch the video).
Image Credit: Interface Focus
Exploring the Damage
From the high-speed video, the team pulled data for each of the 20 tests on the velocity of the arrow prior to impact. Using this and the corresponding mass of the arrow, they calculated the kinetic energy (the energy related to the arrow’s motion) and momentum for each test.
In order to describe the damage caused by a puncture, the scientists examined three things—how far into the gelatin the arrow went (penetration depth), how much of the gelatin was deformed by the arrow but did not tear (pull-in area), and length of gelatin that tore (tear length).
The measures of damage. (a) Penetration depth: length from the edge of the gelatin to the tip of the arrow. (b) Tear length: length along the edge of the arrow where new surface is being created, denoted by the region where gelatin is no longer being pulled in along the arrow shaft, but is torn instead. Both penetration depth and tear length were measured from single frames at maximum depth. (c) Pull-in area: area of material on the impact surface being deformed during penetration by the arrow.
Image Credit: Interface Focus
For each of the 20 cases, they used mathematical techniques to look for correlations between velocity, kinetic energy, and momentum and two characterizations of damage—penetration depth and tear length. Here are the results for the range of velocities (about 18-31 mph) and mass (about 0.6-1.5 ounces) they considered.
Kinetic Energy was by far the best predictor of how effective the arrow was at causing damage. Arrows with higher kinetic energy went further into the gelatin and caused more tearing. This makes sense in light of cutting theory, which says that cutting or tearing a target requires energy. The more energy you start with, the more energy you can put into cutting.
The velocity of the arrow showed no correlation to the penetration depth or the tear length, which may come as a surprise considering that kinetic energy depends a lot on the velocity. However, kinetic energy also depends on mass. You can increase the kinetic energy of an object by increasing its mass or its velocity. This lines up with what happens in the animal world—animals with smaller (lighter) puncturing weapons, like jellyfish and insects, tend to puncture objects at much higher speeds than those with more massive weapons. Both extremes have a lot of kinetic energy.
The velocity did impact the damage in another way, however, which you can see by comparing tests that have the same penetration depth and mass, but different velocities. Arrows with higher velocity deformed the gelatin less near the impact area. This means you can create more damage with fast projectiles because more of the kinetic energy goes into tearing the target and less into deforming it.
The momentum showed a possible correlation with penetration depth, and none with tear length. This was surprising because momentum and stress—the forces a target feels that cause it to deform and crack—are closely related. The researchers suggest that momentum may play a more important role in situations where it is harder for the projectile to puncture the target than it was to puncture the gelatin in their experiments.
Expanding the Framework
Exciting work remains to be done. This research explored a range of masses and speeds, but the range in which animals puncture is much broader for both variables. Does this pattern hold in the extremes? How does the shape of a puncture weapon impact its effectiveness? What effect does the material a weapon is made of have on its ability to cause harm? What can learn from these animals when it comes to designing high-speed cutting devices of our own? The researchers hope that their work has laid a foundation for discussing these issues and opened the door to further studies of this fascinating ability that so many animals depend on for survival.
—Kendra Redmond